The development of radiopharmaceuticals represents a critical intersection of medicine, chemistry, nuclear physics, and pharmaceutical sciences, with preclinical imaging techniques playing an indispensable role in this process. Preclinical imaging facilitates the visualization and quantification of radiopharmaceutical distribution within biological systems, providing invaluable insights into their pharmacokinetics and biodistribution. Imaging modalities, such as Single Photon Emission Computed Tomography (SPECT) and Positron Emission Tomography (PET), together with CT/MRI, enable researchers to visualize radiopharmaceuticals’ distribution and anatomical localization within biological systems in real-time. The importance of preclinical imaging extends beyond mere visualization; it is fundamental for evaluating radiopharmaceuticals’ therapeutic potential and safety profiles. By assessing pharmacokinetics—how a drug is absorbed, distributed, metabolized, and excreted—these imaging modalities provide a comprehensive understanding of how effectively a radiopharmaceutical targets its intended tissues (Sgouros, 2023). This knowledge is crucial for optimizing dosage regimens that enhance therapeutic effects while minimizing potential toxicity (Sgouros, 2023).
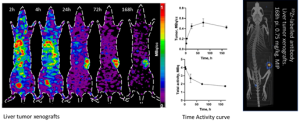
Figure 1: Tumor uptake and Biodistribution of an 89Zr labeled antibody in liver tumor xenograft over the different period
However, the application of preclinical imaging is not without its challenges. Issues such as the scarcity of specific radionuclides for targeted alpha therapy and limitations in current imaging technologies necessitate ongoing research to refine these methodologies. Integrating advanced preclinical models also poses logistical challenges that must be addressed to ensure accurate translation from bench to bedside.
One primary issue is the scarcity of specific radionuclides, particularly those used in targeted alpha therapy (TAT). The limited availability of alpha-emitting radionuclides, such as Actinium-225 and Lead-212, poses substantial obstacles for researchers aiming to develop effective imaging modalities for these agents (Machaba, 2024). This scarcity complicates the ability to conduct thorough preclinical evaluations and may delay the progression of promising therapies into clinical trials.
It is also noteworthy that the investigation of alpha particle-emitting radionuclides presents significant challenges, particularly regarding the mismatch between their energy profiles and ethically justifiable scanning periods for animal subjects. The available options for evaluating alpha emitters’ tissue distribution include specialized imaging techniques (alpha camera systems or SPECT). Furthermore, theragnostic approaches can be employed if there is a uniform distribution between diagnostic and therapeutic agents, enhancing treatment efficacy while mitigating ethical concerns. On this note, some studies deserve to be highlighted. For example, to realize imaging of Actinium-225, the presence of daughter decay can be utilized to produce SPECT by way of capturing 11% of 218 keV emissions produced by Francium-221 and the 26% of 440 keV produced by Bismuth-213 (Yong Du 2022).
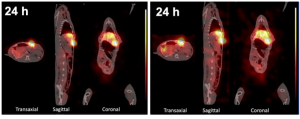
Figure 2: Representative nuclear imaging of a rabbit bearing VX2 hepatic tumor xenograft. The micro-SPECT/CT images were acquired and reconstructed using the Francium-221 energy window (left) and Bismuth-213 energy window (right), respectively (Yong Du et al., 2022)
Furthermore, existing imaging technologies often have limitations regarding sensitivity and resolution. While techniques like SPECT and PET are invaluable for visualizing radiopharmaceutical distribution, they may not be sufficiently advanced to detect low levels of radioactivity or differentiate between closely located tissues effectively. This inadequacy can result in inaccurate assessments of biodistribution and pharmacokinetics, ultimately affecting the interpretation of therapeutic efficacy (Sgouros, 2023). As a result, researchers must continuously seek innovative strategies to enhance imaging capabilities while ensuring that these advancements align with regulatory standards.
Additionally, there is often a lack of standardized protocols concerning preclinical imaging practices across different research institutions. This inconsistency complicates data comparison and interpretation between studies, making it challenging to establish universal benchmarks for assessing radiopharmaceuticals (Olkowski, 2023). The absence of harmonized methodologies may lead to variability in results that could obscure genuine therapeutic effects or safety concerns.
Finally, ethical considerations surrounding animal welfare also present a challenge in preclinical imaging applications. Researchers must balance the need for extensive biodistribution studies with the ethical imperative to minimize animal use and suffering (Hobbs, 2023). Using improved (higher resolution and sensitive) non-invasive imaging techniques that require fewer animals while still yielding robust data is essential, but it remains an ongoing area of research.
Another challenge lies in integrating advanced preclinical models into research protocols. Complex animal models—such as patient-derived xenografts (PDX) or genetically engineered mice—can yield more relevant data regarding human responses; however, these models also introduce logistical difficulties. Variability among individual animals can affect the reproducibility of results, making it challenging to draw definitive conclusions about a radiopharmaceutical’s performance across different biological contexts (Hobbs, 2023). Moreover, ethical considerations surrounding animal welfare necessitate careful planning and compliance with established guidelines.
In conclusion, the continuous evolution of imaging technologies bolsters our capacity to visualize intricate biological processes. It paves the way for innovative therapeutic strategies that improve patient outcomes in nuclear medicine. As we advance into an era of precision medicine and personalized healthcare solutions, the synergistic relationship between preclinical imaging modalities and radiopharmaceutical developments will remain pivotal in effectively addressing emerging health challenges.
References
Olkowski, C., (2023). Preclinical Development in Radiopharmaceutical Therapy for prostate cancer. https://www.sciencedirect.com/science/article/abs/pii/S0001299823000533
Machaba, M., (2024). Do current preclinical strategies for radiopharmaceutical development meet the needs of targeted alpha therapy? https://link.springer.com/article/10.1007/s00259-024-06719-5
Sgouros, G., (2023). Guidance for Preclinical Studies with Radiopharmaceuticals | IAEA. https://www.iaea.org/publications/14818/guidance-for-preclinical-studies-with-radiopharmaceuticals
Hobbs, R. F., (2023). The Role of Preclinical Models in Radiopharmaceutical Therapy. https://ascopubs.org/doi/10.14694/EdBook_AM.2014.34.e121
Du Y., (2022). Preliminary evaluation of alpha-emitting radioembolization in animal models of hepatocellular carcinoma. PLoS ONE. https://doi.org/10.1371/journal.pone.0261982